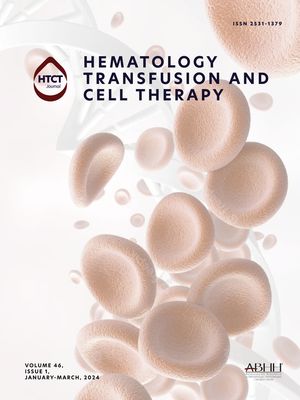
The use of immunotherapy in cancer treatment over the past decade has resulted in significant advances and improvements in cancer patients survival with the use of checkpoint inhibitors. Nevertheless, only a fraction of solid tumors responds to this immunotherapy modality. Another modality of immunotherapy consists of employing cell-based therapy as an adoptive therapeutic modality. That involves distinct modalities of cellular therapies such as CAR T cells (chimeric antigen receptor T cell), TILs (tumor-infiltrating lymphocytes), and TCR T cells. Those treatments have proven effective in hematologic tumors and could have an impact in tumors that do not respond to checkpoint inhibitors. This review aims to outline the rationale, operation, clinical applicability, and results of adoptive cell therapy for patients with solid tumors.
Immunotherapy has made significant progress in the development of cancer treatment in recent years. Major therapeutic strategies include nonspecific stimulation of the immune system, active immunization using cancer vaccines, and adoptive cell transfer immunotherapy. Distinct modalities of cell therapies have been used in clinical trials for the treatment of solid tumors. These modalities consist of CAR T cells, TILs, and TCR T cells (Figure 1). Other modalities such as NK-CARs, gamma-delta T cells, and T cells against neoantigens are under investigation. The use of second-generation anti-CD19 CAR-T cells has displayed exceptional results in clinical trials against hematologic tumors, which led to the approval of the treatment against non-Hodgkin lymphoma (NHL) and acute lymphoid leukemia (ALL-B) in both the US and Europe. However, equal success has not been observed regarding the treatment of solid tumors. Given this limitation, novel technologies and generations of CAR T are under development to overcome those hurdles and allow effective cell therapies to be therapeutic options against solid tumors. Despite many ongoing studies using various strategies and technologies, few studies have shown significant clinical results. Cell therapy is an innovative modality with great potential in solid tumors. However, it has yet to prove its efficacy in solid tumors.1–4
Tumor infiltrating lymphocytes (TILs)Treatment with adoptive cell transfer obtains peri-tumor lymphocytes through the patient's tumor sample, which is resected, fragmented, and cultured in interleukin-2 (IL-2). The lymphocytes grow, destroy the tumors, and within two to three weeks generate pure lymphocyte cultures testable for reactivity. The cultures are expanded until an adequate number is obtained for lymphocyte infusion into the patient. Before lymphocyte infusion, the patient must receive cytotoxic chemotherapy for lymphodepletion. Thus, the patient must fulfill some prerequisites, such as tolerating the chemotherapy, having resectable tumor lesions, and present a clinical condition that allows a waiting period of four to six weeks for the growth of cells in vitro. That strategy has been tested mainly in patients with metastatic malignant melanoma, where significant clinical results were obtained.19 Studies using TILs in other indications are currently in progress.5
The first successful adoptive cell therapy used in solid tumors was the infusion of TILs into patients with metastatic melanoma.6 Malignant melanoma ranks as one of the most immunogenic solid tumors. Therefore, it has been a prototype for investigations of active cancer immunotherapy.7,8 Pioneers in the use of infiltrating lymphocytes in 1988 demonstrated that TIL can induce cancer regression when administered to patients with metastatic melanoma.9 An analysis of 86 melanoma patients treated with TIL followed by high dose IL-2 demonstrated a response rate of 34%. Out of those, five patients (6%) achieved complete response, but in only two patients the responses lasted between 21 and 46 months.10 Since then, there has been an effort to improve these results through modifications in the methodology of TIL generation and selection, as well as changes in treatment regimens prior to TIL.
Recently, the use of TILs targeting specific proteins encoding somatically mutated genes has shown clinical regressions in patients with different metastatic solid tumors, such as malignant melanoma, colorectal, biliary tract, cervical, and breast.11–19
Rosenberg et al. reported a case of metastatic colorectal cancer treated with TILs, which showed a polyclonal response of CD8 + T cells against the KRAS G12D mutation, with objective regression of lung metastases.14 Zacharakis et al. reported the case of a patient with hormone receptor-positive, refractory breast cancer who was treated with TILs reactive against mutant versions of four proteins (SLC3A2, KIAA0368, CADPS2, and CTSB), associated with interleukin IL-2, showing sustained complete regression of metastatic breast cancer.17 Phase II study showed the efficacy of TIL in the treatment of metastatic human papillomavirus-related tumors with objective response in 28% in the cervical cancer cohort and 18% in the non-cervical cohort (anal canal cancer and oropharyngeal cancer).18
The most important clinical study of this treatment in solid tumors was probably the phase 2, multicenter, single-arm study that evaluated the use of Lifileucel in 66 patients with metastatic melanoma refractory to immunotherapy and targeted therapy (if mutated BRAF). The overall objective response was 36%, with two patients showing a complete response, and the disease control rate was 80%. Additionally, the toxicity profile proved safe for patients who were not candidates for immune checkpoint inhibitors due to prior adverse events.19
The advent of TILs therapy may provide a treatment option for patients with metastatic melanoma refractory to previous treatments. Also, that therapy can potentially benefit patients with epithelial cell neoplasms with lower mutation rates that are less responsive to checkpoint inhibitor therapies, such as tumors of the gastrointestinal tract, breast, and ovary. In addition, there are association studies of TILs with imaging techniques to evaluate the effects of neoadjuvant chemotherapy in PET-TIL experiments in the neoadjuvant treatment of breast cancer.20 There are also prognosis assessment studies using imaging systems to determine the rate of immune cell infiltration and its correlation with prognosis.21
CAR-T cellsThe advent of effective methods for gene transfer into T cells provides a new means to create tumor-specific T cells, with the possibility of transferring genes that encode chimeric antigen receptors or T cell receptor genes (TCR).5,22 Tumor-specific T cells can be generated by transferring genes that encode chimeric antigen receptors (CARs). CARs consist of a tumor antigen-binding domain previously fused to an intracellular signaling domain capable of activating T cells. Antigen recognition, therefore, is not HLA restricted, like physiological TCR; instead, it is targeted to native cell surface structures. The binding of the ligand to the chimeric receptor triggers phosphorylation in the cytoplasmic region of the molecule, activating the signaling cascade required for induction of cytolysis and secretion and proliferation of cytokines. The T-cell activation properties of CARs depend on their composition.4 The results obtained with CAR-T cells in hematologic tumors have exerted a significant clinical impact and have changed treatment options. Studies on adoptive cell therapy with CAR T cells directed against the surface glycoprotein CD19 in hematopoietic tumors have resulted in sustained remissions even in patients with recurrent or refractory B-cell malignancies.23,24 Those results led to the approval in the US and Europe of three CAR-T cell therapies targeting CD19 in patients with non-Hodgkin's lymphoma (NHL) and B-cell acute lymphoblastic leukemia (B-ALL).
Driven by the results in hematologic tumors, CAR-T cell therapy development in solid tumors has not shown similar results. It faces several obstacles, starting with the high molecular and clonal complexity of solid tumors, the histopathological characteristics, and their different anatomical locations. Additionally, there are limitations regarding the highly hypoxemic, immunosuppressive tumor microenvironment, inhibition of T-cell function, antigen expression heterogeneity, and immune evasion of the tumor due to antigen loss. Due to the possible similarity in antigen expression in epithelial tissues, the toxicity caused by relative target expression in normal tissues poses another limitation to be overcome by new methodologies. Still, one of the major challenges of the application in solid tumors is identifying tumor-specific surface antigens capable of being recognized by the CAR. That represents a major limitation for the realization of adoptive cell therapy in solid tumors, considering that most of the known "high priority" tumor antigens and most of the neoantigens are intracellular proteins. Therefore, they are only subject to immune surveillance as cell surface peptides, carried by major histocompatibility complex (MHC) molecules.3,25,26 Also, the wide tumor heterogeneity leads to a low-probability scenario in which a target is present in a large proportion of patients with the same solid tumor.
A significant difference in the current CAR T results between solid tumors and hematologic tumors arises from the hurdle in finding an ideal target antigen. That is because, in contrast to hematologic tumors, solid tumors rarely express a tumor-specific antigen. In most solid tumors, a tumor-associated antigen (TAA) is more commonly found, where this antigen is overexpressed in tumors, but also expressed at low levels in normal tissues. That occurs in several TAAs often targeted to solid tumors, such as CEA, ERBB2, EGFR, GD2, mesothelin, MUC1, and PSMA. The lack of tumor antigen specificity increases the potential risk of toxicity, which limits the use of TAAs that despite being overexpressed in the tumor, display expression in normal tissues.27
In addition, solid tumors tend to present a high degree of antigen heterogeneity, often presenting only a subset of cells that express the target antigen. Even when a TAA is uniformly expressed, there is the possibility of antigen loss, antigen leakage, or even negative regulation of antigen when the target antigen disappears from the surviving tumor.27–33 Bicistronic or bispecific CARs, which recognize two antigens, were created to address antigen heterogeneity and the threat of antigen loss.34–37
Considering that solid tumors present high heterogeneity - and evidence of antigen leakage - another strategy that may enhance the efficacy of CAR T cell therapy is the concept of epitope spreading, in which an epitope different from the original target antigen becomes the target of endogenous T cells. Those epitopes arise from tumor lysis of some cells, leading to the release of these new tumor-specific antigens or epitopes, which then are presented by antigen-presenting cells and targeted to endogenous TILs, thereby generating a secondary immune response. There is evidence of this spread in vaccine and CART therapy studies in tumors such as malignant melanoma, lung cancer, and pancreatic cancer.38–40
Several strategies have been studied aiming to improve CAR T-cell efficacy in solid tumors. Those strategies include either active elimination of cytokines and chemokines to increase T-cell activity or in combination with other treatments that increase anti-tumor T-cell activity, such as checkpoint inhibitors (anti-PD1).11–14 Novel next-generation CAR T modalities are under study to increase the anti-tumor capacity of these cells and make them more resistant to the tumor microenvironment. CAR-T cells that have PD-1 knock-out, secrete cytokines (e.g., IL-12, IL-18), or have a higher percentage of less differentiated T cells (Tscm, Tcm) may exhibit more pronounced anti-tumor effects and have an increased chance of showing high efficacy.41–44
TCR-T cellsT cell specificity is TCR-mediated, consisting of α and β chains that genetically determine T cell antigen specificity in a clonotypic manner. The α/β heterodimer recognizes peptides presented by molecules encoded by the major histocompatibility complex (MHC), referred to as human leukocyte antigens (HLA). Thus, antigen recognition is restricted by each individual's HLA. One way to improve tumor recognition by autologous T cells is to introduce preselected TCR genes. The ability of the TCR to recognize a tumor cell is restricted by the HLA antigens expressed by the tumor. Among the methods for gene transfer to T cells, retroviral vectors are the most commonly used, but other systems, including adenoviral and non-viral systems, are also used.
Only about 1% of total cellular proteins are expressed on the cell surface. A large number of potential tumor antigens are not available to a CAR T cell.45 Therefore, the construction of a TCR-T that recognizes antigen in combination with the MHC enables the recognition of extra- and intracellular antigens. Recent studies have identified several new tumor-associated antigen (TAA) candidates that could be amenable to TCR-T cell therapy with promising results at the preclinical and clinical levels.27
The NY-ESO-1 antigen has been prominent in that scenario. That is a type of antigen from the group termed cancer-testis antigens (CTA), which are proteins that play a role in immune maturation, restricted to human germ cells, expressed in fetal testicular germ cells, ovarian cells, and the placenta, with low expression in normal somatic cells in the adult. However, these antigens can be re-expressed in various malignancies, as in the case of NY-ESO-1, a CTA well known for re-expression in numerous cancers.46 In 2016, Patel et al. showed success in an in vivo myeloma study with a CAR/TCR hybrid that recognizes the NY-ESO-1 antigen in the context of HLA-A2.47 Sarcoma studies have been prominent, showing homogeneous expression of NY-ESO-1 in several sarcoma subtypes, notably Myxoid/round cell liposarcoma (MRCLS) and synovial sarcoma (SS).46,48 In the study conducted by Robbins et al., which initially treated six patients with SS, all with HLA-A*0201 haplotype and high expression of NY-ESO-1, four patients achieved objective response lasting 5–18 months.49 In light of this result, an expanded cohort was conducted with 12 patients with SS, among whom 5 received the addition of the NY-ESO-1 peptide vaccine to enhance antitumor immunity. In this new cohort, seven patients achieved an objective response, and the objective response rate of NY-ESO-1 TCR in SS was 61%, with a response lasting from 3 to 47 months.50 Another study for the treatment of patients with synovial sarcoma used TCR T cells against the MAGE-4 target and also showed significant initial results with 44% clinical response with a median response duration of 28 weeks.51
Additional modalities of cell therapyAnother CAR modality under investigation in solid tumors is NK CARs, which correspond to the expression of the chimeric receptor on Natural Killer cells, other than on T cells. The studies are still incipient, and the sole clinical trial published up to date was a phase 1/2 study. That study evaluated umbilical cord cell-derived CAR NK cells expressing anti-CD19, interleukin-15, and caspase 9, with the help of retroviral vectors, to treat patients with CD19-positive hematologic tumors. No cases of graft-versus-host disease were observed, despite the absence of HLA compatibility in two patients and partial compatibility in the other nine patients. Out of 11 patients, eight subjects responded to treatment, with seven having a complete response. However, those initial data are to be used to understand the safety of this technique, but we cannot consider it as evidence for the effectiveness of this treatment modality.52 The idea of this investigation arises from the perspective that perhaps the NK CAR can overcome some barriers of the T CAR, especially in solid tumors, considering that the NK hardly recognizes healthy cells since they have the MHC, which binds to the inhibitory NK receptor. Thus, that technique is under study as a promise to reduce the side effects caused by cell therapy in solid tumors since they most commonly share antigens with cells from other than tumor tissues. In addition, another advantage of that technique may stem from the fact that it does not require prior sensitization and HLA-matching. Thus, it could be universally produced for given antigens, thus becoming an 'off-the-shelf' cell therapy. That should provide faster treatment, without the need for weeks of waiting for CAR production, and without the risk of insufficient lymphocytes obtained at apheresis in patients who had already received several myeloablative therapies.53 Other modalities also under investigation include the use of NKT-invariant cells, gamma-delta T cells, and T cells that use neoantigens as targets. NKT-invariant cells function like T cells, display TCR on their surface but recognize glycopeptides instead of MHC molecules, and thus potentially used in the allogeneic “off the shelf” setting. Due to a potential advantage in infiltrating tumor tissues, perhaps this may be an alternative to treat solid tumors. Nevertheless, the low availability of those cells in circulation may be a limiting factor regarding their clinical use.54 Gamma-Delta T cells are also present in small quantities in circulation. However, the expression of gamma-delta TCR may lead to an advantage in recognizing a varied range of antigens and be activated in a completely distinct way from conventional T cells.55 The use of neoantigens as targets for cell therapies is a new modality currently under investigation in clinical trials. The advantage of this therapy lies in the use of highly tumor-specific targets, providing a highly personalized treatment with high specificity. The time required to identify neoantigens and manufacture this product may limit this therapy.56 Clinical studies are underway to validate those novel therapies. Thus, we have to wait for the upcoming results for insights into the best implementation strategy.
ConclusionCAR T cell therapy development has revolutionized the treatment of patients with hematologic malignancies. Nevertheless, the data in solid tumors have not been as expressive,57 probably due to multifactorial causes, including low amounts of membrane antigens recognizable by immune cells, tumor antigenic heterogeneity, complex tumor cell evasion mechanisms, and immunosuppressive tumor microenvironments. Therefore, several strategies are under study to improve the efficacy of adoptive cell therapy in solid tumors. Those strategies include exploring new targets, using safety switches, incorporating cytokines and chemokines to increase T-cell activity and infiltration, exploring a combination with other treatments, and investigating the possibility of using other immune cells such as CAR-NK, NKT-invariant, and gamma delta T cells.58–61
Nonetheless, the results obtained have not yet provided solid scientific evidence that would allow us to introduce these techniques outside of the context of clinical research for solid tumors. Up to date, the most advanced cell therapies with the most promising data are TILs for the treatment of melanoma patients. However, the clinical trial data are not yet definitive and require regulatory approval before commercialization. The results of ongoing studies aimed at improving safety and efficacy through further refinement of these techniques and new technologies remain eagerly awaited.