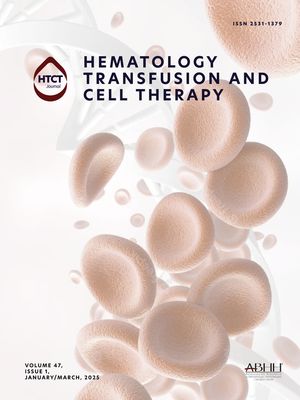
Chimeric antigen receptor T cells (CAR-T), especially against CD19 marker, present in lymphomas and acute B leukemia, enabled a revolution in the treatment of hematologic neoplastic diseases. The manufacture of CAR-T cells requires the adoption of GMP-compatible methods and it demands the collection of mononuclear cells from the patient (or from the donor), generally through the apheresis procedure, T cell selection, activation, transduction and expansion ex vivo, and finally storage, usually cryopreserved, until the moment of their use. An important aspect is the quality control testing of the final product, for example, the characterization of its identity and purity, tests to detect any contamination by microorganisms (bacteria, fungi, and mycoplasma) and its potency. The product thawing and intravenous infusion do not differ much from what is established for the hematopoietic progenitor cell product. After infusion, it is important to check for the presence and concentration of CAR-T cells in the patient's peripheral blood, as well as to monitor their clinical impact, for instance, the occurrence of short-term, such as cytokine release syndrome and neurological complications, and long-term complications, which require patient follow-up for many years.
The manufacture of cell products for clinical use is still in its early phases of development. One example is the production of genetically modified cells, the chimeric antigen receptor T cells, with specificity against antigens expressed in tumors,. For cell therapy products, the evolution of phase 3 trials until the approval of the final product, or its platform of manufacture, by governmental regulatory agencies is estimated to be at least 15%, whereas for pharmaceutical products the index is almost 50%.1 The manufacture of a cell product requires additional steps in relation to the manufacture of a molecular agent, such as selection, purification, possible modification of their characteristics, formulation, preservation and, lastly, distribution of cells of interest. It might as well require the performance of laboratory assays to evaluate any possible alteration cells undergo after their administration, once the cells are “living drugs” that can acquire characteristics not predicted initially or lose the characteristics expected to be conserved. Small changes in the manufacture process may cause significant alterations in the final product, and then the product quality control offers big challenges to cellular therapy laboratories and regulatory agencies.
One of the critical aspects in the production of a cell product concerns its origin, whether autologous or allogeneic, even if the purpose is the same. Safety criteria tend to be more stringent in cases of allogeneic use of the cell product, mainly due to a higher risk of transmission of infectious agents from the donor to the recipient. Therefore, the donor of the cells must be subjected to a series of laboratory assays to detect the most commonly transmitted agents, what depends on the region or country where the donation takes place. Generally, the mandatory tests for blood donation are the same for the donation of other cell types or tissues. However, the final product must be also tested for infectious agents that may have contaminated the product during its manufacture phase, for example, bacteria, fungi, and mycoplasma. An important aspect for product safety is the method of cell culture, in an open or closed system. Certainly, closed systems ensure greater safety to the manufacture process, because they reduce substantially the risk of contamination by infectious agents. Moreover, closed systems, as bioreactors, allow to scale up and gain time in the process of expansion of the number of cells of interest, critical aspects in cellular therapy, even in case of an autologous CAR-T cell product, in which the patient often shows a clinical picture that asks for agility in the treatment administration. Apart from the issue of sterility, the final product must be also tested for the presence of residues from the manufacture process, for example, the amount of microbeads in the CAR-T cell product.
Likewise, the final product has to be assessed regarding its basic characteristics, such as the cell type of which it is composed (identity), and the purity grade, as well as the potency it is expected to have; after all, its potency largely determines its clinical utility. This last item is particularly difficult to evaluate in a cell product, mostly because there are not assays available, as a rule, which could precisely predict the effects the cell product will have in vivo.
Collection of lymphocytesThe collection of lymphocytes for CAR-T cell manufacture is generally performed through leukapheresis.2 Most institutions collecting hematopoietic progenitor cells (HPC) are competent to collect lymphocytes, once this procedure does not differ much from the technical point of view. In addition, the collection of lymphocytes does not require their mobilization to peripheral blood (PB), what is a rule for HPC. The yield of the apheresis collection is approximately 40-50% of circulating mononuclear cells (and lymphocytes). The ultimate goal is to obtain at least 1–2 × 109 CD3+ cells, which may not be easy in a significant number of patients with lymphopenia, at a great extent, because they have undergone several lines of chemotherapy treatment. Lymphocyte concentrations lower than 300/μL may make the collection unviable. Furthermore, after recent chemotherapy, the first lymphocyte subpopulation to increment in PB is NK cells and not CD3+. With a concentration of lymphocytes of 500–600/μL, the processing of three or more blood volumes may be necessary. In our experience, the yield of the collection is about 50%, in other words, half of mononuclear cells that go through the apheresis machine is collected. For example, a patient with a lymphocyte concentration of 500/μL in PB, and 300/μL of CD3+ cells, would have a collection of 150/μL of this latter subpopulation, in other words, 1.5 × 108 per liter processed. Therefore, in this case, at least 10L of PB must be processed to reach the minimal goal (1.5 × 109 CD3+ cells), but preferably 15–20 L corresponding to more than 3 blood volumes to, in addition to ensuring the minimal dose, obtaining a “surplus” that could be beneficial for the confection of the final product at an “optimal dose”. For this reason, it is important to quantify CD3+ lymphocytes in PB before the beginning (or at the beginning) of the leukapheresis procedure in order to program the processing of the volume that allows to reach the aim of the collection.3,4
The adverse effects of leukapheresis are usually mild and short-lived, such as hypocalcemia, which causes mainly paresthesia in extremities. A problem could be the inadequate peripheral venous access for the apheresis procedure in a large number of patients, who for this reason need the insertion of a central vein catheter with the its inherent risks.5
Concentration and enrichment of T cellsThis step aims to reduce cell contaminants that could compromise the quality of the final product. Among contaminating cells with negative effect on the processing are monocytes, granulocytes, platelets, and erythrocytes. For example, monocytes and granulocytes inhibit the expansion and transduction of T cells.
Manual or automatic washing with saline removes the remaining platelets and plasma. Subsequently, there is the step of mononuclear cell sorting, in Ficoll® or LSM®, or through equipment like Sepax II or Rotea, for instance. However, after these two steps, a large number of monocytes often remains, but it can be removed by the method of surface adhesion or elutriation. An alternative to the mentioned methods is immunomagnetic separation in which it is possible to retain the cells of interest and eliminate all other cells that do not express the marker of the cell to be retained (CD4+/CD8+, in case of CAR-T).6,7 The contamination by NK cells may be a problem, so some authors recommend their removal whenever their number exceeds 10% of the total. The final product of this step has to be assessed for its immunophenotypic characteristics. This step can be finalized with the cryopreservation of cells to be stored until the moment of moving to subsequent steps, or then following to the activation and transduction steps.8
Activation of T cellsThe activation of T cells is a critical phase of the manufacture process of CAR-T cells, setting up a prerequisite for the transduction step. The activation of T cells is normally given by their interaction with antigen presenting cells, such as, for example, dendritic cells (DC). Since the culture of T cells with DC is laborious, other methods that mimic this interaction have been put into practice, such as the activation by antibodies. The activation is enabled by adding the anti-CD3 antibody (or its Fab fragment), in combination with the anti-CD28 antibody, to cell suspension, immobilized (soluble antibodies seem to be less effective) in a substrate, for example, immunomagnetic microbeads (ex. Dynal Dynabeads; Human T-activator). Magnetic microbeads have 4.5 μm in diameter and are covered with activating antibodies. The problem of this strategy is the need to remove, using a magnet, almost the total amount (<100 microbeads for 3 × 106 cells) of the product microbeads before its infusion, a relatively laborious procedure requiring, as a quality control assay, their quantification in the final product.9 Other substrates can be used rather than microbeads, whose main advantage would be not going through the procedure of removal, such as for example StreptActin associated with Fab fragments of anti-CD3/CD28 antibodies that can be removed by washing the cell suspension or a biodegradable nanomatrix to which the referred antibodies would be linked.8,10
TransductionThe process of transduction demands the employment of a viral vector, lentivirus or retrovirus, or a non-viral vector, as transposon/transposase. Retroviruses were the first to be used, however, lentiviruses are currently preferred for safety reasons and because they offer the advantage of also transducing non-dividing cells.11
The process of transduction is given by the incubation of activated T cells with the viral (or non-viral) vector, which shelters the transgene (the fragments corresponding to the CAR sequences, to the co-stimulating molecule B4-1BB or CD28 and to the signaling molecule CD3ζ) to be introduced into cells. The use of non-viral vectors (e.g., sleeping beauty and piggyBac) is considered relatively simple, although it is less efficacious in terms of transduction and poses the risk of random integration of the transgene and, thus, the risk of insertional mutagenesis. On the other hand, the use of viral vectors shows much higher cost once it depends on a laborious production process, in addition to requiring a clean room infrastructure and the adoption of safety measures, both of environmental nature and in terms of the final product.9
Cellular expansionAfter the transduction step, CAR-T cells have to go through a process for expanding their number before infusion in the patient. This step can be performed in T flasks for cell culture, in plastic bags, bioreactors or in the system CliniMACS Prodigy (Miltenyi BioTec). T flasks are characterized as an open system with inherent risks and their several units have to be manipulated individually for the culture process of a single batch of cells, which makes it somewhat impractical, especially if one intends to scale up the production. Bioreactors offer many advantages, such as relying on a closed system of culture, meeting the microbiological safety requirements, in addition to allowing the handling of a single piece of equipment, as the Prodigy system, which enables to conduct virtually all the manufacture process in a single closed system, so having the infrastructure of a high classification clean room is not necessary. Nonetheless, this system is relatively expensive, because, apart from the equipment itself, all the inputs used in it are of exclusive nature, in other words, they do not allow adaptations to local realities.8,10
CryopreservationThe cryopreservation of CAR-T cells is not a mandatory step in every case; however, this procedure may be needed for logistic reasons, as for example to gain enough time in order to prepare the patient for the infusion of the cell product or to meet the regulatory standards regarding the laboratory assays of quality control. Cryopreservation is also needed when one needs to send the cell product to a different service, including abroad. On the other hand, the urgency in the use of CAR-T cells for hematologic neoplasias has made their fresh use more frequent, skipping the cryopreservation step, in spite of their relatively reduced time of stability when stored in their liquid form at refrigerator temperatures, and having higher probability for the growth of contaminating microorganisms.
So far, the effects of the cryopreservation process over CAR-T cells have not been fully known (including thawing). There are two major remarks related to this issue in the setting of CAR-T cell manufacture; the first one concerns its impact on the quality of T cells, mainly its effects on the efficacy of the subsequent steps of transduction and expansion, and the second one is about the impact of CAR-T cell cryopreservation on their viability and function in vivo.
When the starting product is cryopreserved, the number of viable cells at the onset of processing is significantly lower to that observed in the fresh product, nevertheless, the capacity for expansion, the efficacy of transduction, the percentage of CD3+ cells and the CD4:CD8 ratio of the final product did not differ between the two types of initial product, cryopreserved and fresh. Moreover, no influence upon the storage time of the cryopreserved product has been demonstrated in relation to the parameters listed above.12 The same authors showed that the products that underwent double cryopreservation (before the onset of processing and after the completion of CAR-T cell manufacture) had greater recovery of CAR-T cells (final) than mononuclear cells (initial). Another important finding was that there were no differences in terms of clinical outcomes with the infusion of the fresh product and the cryopreserved product at the same cell dose. Lastly, the rate of failure in the production of CAR-T cells did not differ between the groups with initial fresh product or cryopreserved product.12 Other group, though, suggested that the capacity to secret interleukin-2, tumor necrosis factor-alpha and interferon-gamma, was reduced in the previously cryopreserved cell products.13 Another retrospective (phase 1/2) trial, in patients with non-Hodgkin lymphoma, also suggested that there is no difference in terms of clinical outcomes between the group that received cryopreserved product (n = 15) and fresh product (n = 8), for example, the clinical response rate and occurrence of cytokine release syndrome and neurotoxicity were similar in both groups.14
Before cryopreservation, the apheresis product should be stored between the temperatures of 2 and 8°C, so that viability is kept elevated for at least three days, whereas between 10 and 25°C a significant drop in viability occurs within only 24 h of storage.15 The cryopreservation of CAR-T cells requires the use of one or more cryoprotective agents, including dimethyl sulfoxide (DMSO), mostly at the final concentration from 5% to 10%. Panch and colleagues used DMSO at the final concentration of 5% associated with hydroxyethyl starch at 6% and albumin at 4%. Thawing was performed by water bath at 37°C, followed by dilution with Plasma-Lyte A containing heparin 10 U/mL.12 Freezing speed must be slow (1–3°C/min) and the thawing process must be fast. Freezing with the use of equipment that allows to freeze at a programed temperature has been more often employed, once it ensures greater safety to the process; however, some groups have frozen CAR-T products at -80°C, in a mechanic freezer. This last method mentioned enables to reach the freezing speed of 1–3°C/min as long as the temperature for the standard volume of the CAR-T bag is validated, which in this case should have approximately 100 mL (lower volumes are exposed to higher freezing speeds, what could compromise the viability of the cells). It is recommended that the storage of the CAR-T cell product is performed at temperatures lower than -150°C, preferably immersed, to prevent the possibility of cross-contamination in the phase of steam of the liquid nitrogen tank.8,16
Thawing and infusionThe thawing process of the CAR-T cell product must be fast, for example, by water bath at 37°C, occasionally at the bedside, followed by the infusion with no further manipulation or by infusion preceded by dilution of cells with compatible solutions. The advantages of dilution are basically two: decreasing the concentration of DMSO to which cells will be exposed until their effective infusion (which should last about 15–30 min) in order to reduce the toxicity of DMSO to the cells and maybe to prevent the formation of cell lumps after thawing (it is believed that the solution containing citrate offers this potentiality). Moreover, dilution could enable a smaller loss of cells in the circuit in the case of a low volume final product.
The Figure 1 describes the main steps of CAR-T cell manufacturing process and examples of available technologies and devices.
II- Quality controlThe quality control of a cell product aims to evaluate its two key aspects, which are its intrinsic characteristics associated with the expectation about its efficacy and the safety issues, both for the patient and for the manufacture personnel and environment. For this reason, all the topics of quality control discussed below fulfil these aims (Table 1).
Quality control parameters and assays of the CAR-T cell product.
The identity of the cell product is determined through the immunophenotypic characterization of the cells within it. In the case of CAR-T cell product, it is required to determine the amount of CD3+ cells as well as CD4+ and CD8+ or memory cells subpopulations. It is also indispensable to quantify the cells presenting the intended genetic modification or its results, for example, CAR-T expressing the receptor for CD19 marker, which even provides information about the efficacy of the transduction process.
PurityIn addition to the cells of interest, it is important as well to evaluate the content of “contaminating” cells, as the populations of NK and B-lymphocytes and also monocytes. Likewise, non-cellular impurities must be identified, such as endotoxin. Ideally, the tools used for genetic modification should not be present in the final product or, whether present, their amount should be significantly decreased.
ViabilityThe viability of cells must be determined immediately before the product cryopreservation, or before its infusion, in case of a fresh product. The most applied methods are vital stain exclusion, such as Trypan blue, or the count through flow cytometry of cells stained with 7-aminoactinomycin D (7AAD). It is considered that the viability has to be ≥ 70%.
PotencyEvaluating the potency of the cell product is recommended, mainly when it comes to a phase 3 clinical trial (compassionate use or phase 1 clinical trial do not require this evaluation), despite the difficulties in extrapolating possible results obtained in animals or assays in vitro for a clinical treatment situation of a patient. In case of CAR-T cells, the cytotoxicity assay of target cells (in general, it is not possible to use autologous target cells, so that line cells expressing the target marker can be used) might offer relevant extrapolating information, at least in parts, to the clinical setting. However, it is expected that CAR-T cells undergo an expansion process in vivo, in a magnitude difficult to predict, a phenomenon that might increase their potency in vivo in addition to the potency one could predict through in vitro assays or even in animal model. It can be a direct assay, preferably quantitative (it can also be qualitative), for example, the percentage of effectively dead target cells (cytotoxicity), but it can be indirect as well, for instance, the quantification of cytokines (ex. INF-α) released after CAR-T cell stimulus by target cells.
SafetyThe safety of the cell product is one of the foundations of the quality control of which the cell product is an object. The risk posed by a product of genetically modified cells depends on the type and origin of cells, type of vector used or genetic modification method, manufacture process, content of contaminating cells, and non-cellular elements and their purpose of use. We will address here the two main topics related to the safety of the CAR-T cell product: the detection of microbiological contamination and proof of absence of replicating virus in the final product (obviously, in case of using a viral vector). However, there is still a long-term safety issue, widely necessary because of the administration of genetically modified cells, whose adverse effects are not fully understood yet. Therefore, the quality control of a CAR-T cell product does not end with its administration. Patients should be followed up for years to come with the purpose of evaluating the onset of a late complication, such as another neoplasia.
Microbiological contamination can be assessed through methods for the culture of bacteria or fungi in cell suspension samples, associated or not with methods such as Gram staining and search for mycoplasma. An issue in terms of microbiological culture is the absence of validated systems for a cell product (they have been validated only for blood). In general, a culture automated system is used, such as Bactec (Becton Dickinson) or BacT/ALERT 3D (bioMérieux), which should be locally validated for the CAR-T cell product. In Brazil, a culture for aerobic and anaerobic bacteria and fungi is mandatory and, in the case where the product required “extensive manipulation” (RDC 508, May 27, 2021),17 such as it happens with the CAR-T cell product, laboratory testing is also mandatory for the detection of mycoplasma “in samples of the product after processing and before cryopreservation, before and after the addition of cryoprotectants”. The detection of mycoplasma can be performed using the PCR (polymerase chain reaction) method or the MycoAlert assay (Lonza).8
It is still necessary to conduct assays for the detection of replicating viral vectors in the final product, unless their absence has been absolutely established in the starting material and the risk of occurrence has been mitigated during the manufacture process.
StabilityThe integrity and functionality of cells have to be evaluated, particularly to define their shelf life when cryopreserved. In the beginning of the production of CAR-T cells is reasonable that this information is not available yet, nevertheless, a plan to evaluate stability must be implemented.
ConclusionsCAR-T cells are a watershed in the treatment of hematologic neoplastic diseases. Their manufacture requires the adoption of GMP-compatible methods, which demand appropriate facilities, qualified personnel, and establishment of operational procedures for all the process steps, from the collection of mononuclear cells until their administration. The clinical result largely depends on the stringent control of each step of the product manufacture and it goes beyond their infusion in the patient.